X-ray fluorescence (XRF) spectrometers are powerful analytical tools used to identify and quantify the elemental composition of a wide range of materials. This non-destructive technique utilizes the principle of X-ray fluorescence, where a material emits characteristic X-rays when bombarded with high-energy X-rays. By analyzing the emitted X-rays, scientists can determine the elemental makeup of the sample with high accuracy.
There are two main types of XRF spectrometers: Wavelength Dispersive X-ray fluorescence (WDXRF) and Energy Dispersive X-ray fluorescence (EDXRF). Each type offers distinct advantages and disadvantages, making them suitable for different analytical applications.
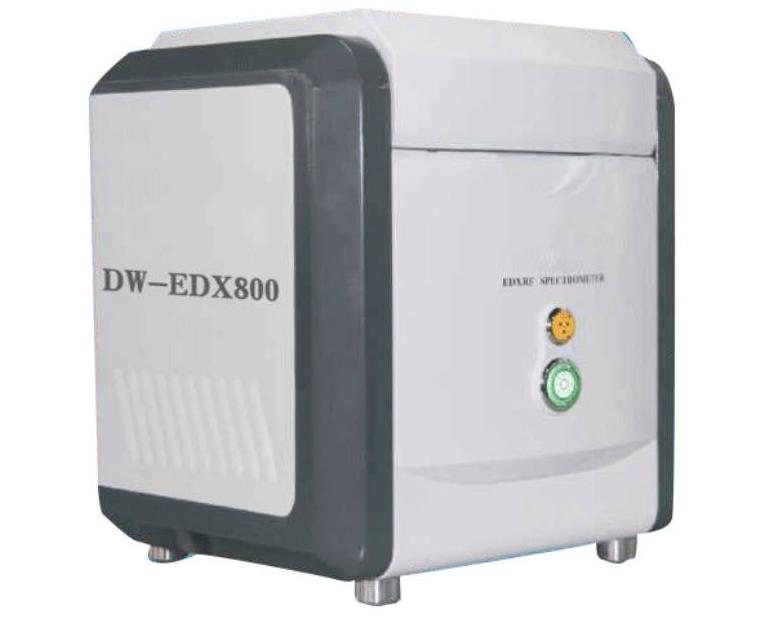
Wavelength Dispersive X-Ray Fluorescence (WDXRF) Spectrometer
Principle of Operation: Separating X-rays by Wavelength
WDXRF spectrometers function by meticulously separating the emitted X-rays from the sample based on their specific wavelengths. Here’s a breakdown of the process:
- Excitation: High-energy X-rays from the source bombard the sample. These X-rays knock out electrons from their inner shells within the atoms of the sample.
- Emission of Fluorescent X-rays: To fill the vacancies created in the inner shells, outer-shell electrons jump down, releasing energy in the form of fluorescent X-rays. These fluorescent X-rays possess energies unique to the elements present in the sample.
- Crystal Diffraction: The heart of WDXRF lies in its crystals. These crystals have a highly ordered atomic structure that acts like a diffraction grating for X-rays. When the emitted X-rays from the sample strike the crystal at a specific angle, only X-rays with a particular wavelength diffract and emerge in a specific direction. This is based on Bragg’s Law, which relates the wavelength of X-rays to the atomic spacing within the crystal.
- Wavelength Separation and Detection: By precisely positioning multiple crystals at strategic angles, the WDXRF spectrometer can separate the emitted X-rays into distinct beams, each containing a specific wavelength corresponding to a particular element. These diffracted X-ray beams then interact with dedicated detectors. Each detector measures the intensity of the X-rays at its specific wavelength. The intensity of the detected X-rays is directly proportional to the concentration of the element in the sample.
Advantages of WDXRF: Superior Resolution and Broad Elemental Range
The unique crystal diffraction approach in WDXRF offers several advantages:
- Exceptional Resolution: Due to the precise separation of X-rays by wavelength, WDXRF achieves outstanding resolution. This allows for the clear distinction between elements with very close atomic numbers, which can be challenging for other techniques.
- Highly Accurate Quantification: The precise measurement of X-ray intensity at each wavelength translates to highly accurate quantification of the elemental abundance within the sample. This makes WDXRF a valuable tool for applications requiring precise knowledge of element concentrations.
- Wide Range of Detectable Elements: WDXRF spectrometers have the capability to analyze a vast spectrum of elements. They can detect light elements like magnesium with low atomic numbers, all the way up to heavy elements like uranium with high atomic numbers. This versatility makes them suitable for a wide range of applications in various scientific fields.
Disadvantages of WDXRF: Cost, Complexity, and Analysis Time
While powerful, WDXRF spectrometers also have some limitations:
- Higher Cost and Complex Design: The intricate design involving multiple crystals and detectors makes WDXRF spectrometers more expensive compared to EDXRF. The operation and maintenance of these instruments often necessitate personnel with specialized training and expertise in X-ray diffraction and instrument operation.
- Slower Analysis Times: Precise crystal positioning and detector alignment are crucial for optimal performance in WDXRF. This can lead to longer analysis times compared to EDXRF, where analysis is often faster due to the absence of complex crystal adjustments.
Energy Dispersive X-Ray Fluorescence (EDXRF) Spectrometer
Principle of Operation: Direct Energy Measurement
EDXRF spectrometers employ a simpler yet effective approach for X-ray analysis. Unlike WDXRF, they forego crystal diffraction and instead utilize a single, solid-state detector to directly measure the energy of the emitted X-rays from the sample. Here’s a breakdown of the process:
- Excitation: Similar to WDXRF, the process begins with high-energy X-rays from the source exciting electrons within the atoms of the sample. This excitation compels electrons to jump to higher energy levels.
- Emission of Fluorescent X-rays: As the excited electrons return to their ground state, they release energy in the form of fluorescent X-rays. These X-rays possess specific energies that are characteristic of the elements present in the sample.
- Energy Detection and Analysis: The core component of EDXRF is the solid-state detector. This detector directly measures the energy of each incoming X-ray. By analyzing the distribution of these energies, called the energy spectrum, the EDXRF spectrometer can identify the elements present in the sample. Each element has a unique fingerprint on the energy spectrum, corresponding to its characteristic X-ray energies.
Advantages of EDXRF: Portability, User-friendliness, and Speed
The single-detector design of EDXRF offers distinct benefits for specific applications:
- Compact, Portable, and User-Friendly Design: Compared to WDXRF’s complex setup with multiple crystals and detectors, EDXRF boasts a more compact and lightweight design. This portability makes them ideal for field applications where analysis needs to be conducted on-site. Additionally, the simpler design translates to easier operation, requiring less specialized training compared to WDXRF.
- Faster Analysis Times: The absence of intricate crystal positioning and detector adjustments in EDXRF significantly reduces analysis time. This enables rapid analysis of samples, leading to faster turnaround times for results.
Disadvantages of EDXRF: Lower Resolution and Limitations with Lighter Elements
While offering portability and speed, EDXRF has limitations in areas where high precision is crucial:
- Lower Resolution and Accuracy: Since EDXRF relies on a single detector to measure all X-ray energies simultaneously, the resolution is lower compared to WDXRF. This can make it challenging to distinguish between elements with very close energy levels, as their X-ray peaks might overlap on the energy spectrum. Additionally, the accuracy of elemental quantification might be slightly lower than WDXRF.
- Limitations with Lighter Elements: Lighter elements emit X-rays with lower energies. These lower energy X-rays can be masked by background noise or by the presence of heavier elements in the sample. This can lead to reduced sensitivity for detecting and quantifying lighter elements.
Applications of XRF Spectrometers
X-Ray Fluorescence (XRF) spectrometers, both WDXRF and EDXRF, serve as powerful tools in a wide range of scientific and industrial applications. Their ability to determine the elemental composition of materials makes them invaluable across various disciplines. Here’s a closer look at some key applications:
1. Geochemistry and Material Science:
Geologists and material scientists leverage XRF to identify and quantify the elemental composition of rocks, minerals, and other materials. This information provides crucial insights into the formation processes of geological features, the properties of materials, and the identification of potential resources. For instance, XRF analysis can help determine the presence of valuable elements in ores or identify the specific minerals present in a rock sample.
2. Environmental Analysis and Pollution Monitoring:
XRF spectrometers are used extensively in environmental monitoring to analyze soil, air, and water samples. They can effectively detect and quantify the presence of harmful contaminants like lead, mercury, arsenic, and other heavy metals. This information is vital for environmental protection agencies to assess pollution levels, track environmental changes, and implement effective pollution control strategies.
3. Forensics and Art Authentication:
In forensic investigations, XRF plays a crucial role in analyzing trace elements present in gunshot residue, paint chips, glass fragments, and other materials collected from crime scenes. By comparing the elemental composition of these materials with potential sources, investigators can establish links between suspects and crime scenes, aiding in criminal investigations.
Unveiling the Authenticity of Artworks: XRF analysis is a valuable tool in art authentication. It helps determine the elemental composition of pigments and materials used in paintings and sculptures. This information can be used to verify the authenticity of artworks by comparing the elemental makeup with known materials used by specific artists or during particular historical periods.
4. Industrial Process Control and Quality Assurance:
XRF technology plays a critical role in various industries for process control and quality assurance. For example, in the electronics manufacturing industry, XRF ensures that electronic components have the correct elemental composition for optimal functionality. Similarly, in the cement industry, XRF is used to monitor the raw materials used in cement production and the final product composition, guaranteeing consistent quality and meeting specific standards.
5. Archaeology and Cultural Heritage Preservation:
Archaeologists utilize XRF analysis to study the elemental composition of artifacts and pottery shards unearthed from archaeological sites. This information provides valuable insights into the materials used by past civilizations, their trade routes, and the technological advancements they possessed. By understanding the elemental makeup of artifacts, archaeologists can gain a deeper understanding of past cultures and societies.
XRF analysis also plays a vital role in cultural heritage preservation. It can help identify harmful elements present in artifacts that can cause degradation over time. This information allows conservators to develop appropriate strategies for preserving cultural artifacts and ensuring their longevity for future generations.
Conclusion
X-ray fluorescence (XRF) spectrometry offers a powerful and versatile technique for elemental analysis. The two main types, WDXRF and EDXRF, cater to different analytical needs. WDXRF provides superior resolution and accuracy, making it ideal for applications requiring precise elemental quantification. EDXRF, on the other hand, offers portability, user-friendliness, and faster analysis times, making it suitable for field applications and quick screening.
The choice between WDXRF and EDXRF depends on the specific requirements of the analysis. Both types of spectrometers play a crucial role in various scientific disciplines and industrial applications. As XRF technology continues to evolve, we can expect even greater advancements in its capabilities, leading to further breakthroughs in diverse fields.